Here we provide a synthesis of the main findings of this project and key fields of some core numerical experiments (eg., nudging simulations in Ding et al. 2022) supported by the project.
Project
NSF/OPP-Arctic Natural Sciences: Arctic sea ice variability: Remote drivers and local processes
(1744598, PI: Qinghua Ding, Co-PI: Axel Schweiger, 2018-2022)
Summary:
A series of studies supported by our project collectively reveals the complex nature of climate change in the Arctic and the role of internal atmospheric variability in masking or strengthening anthropogenically driven Arctic Amplification on interannual-to-multi decadal time scales over the past decades. This internal variability, resulting from a strong coupling between the atmosphere and sea ice in summer, is found to account for about 30 to 40% of the September sea ice decline over the past decades.
This atmosphere-sea ice coupling process is characterized by a multi-decadal summer large-scale circulation trend toward an anticyclonic anomaly from 1979 to 2020 over Greenland and the Arctic, which is suggested to be able to modify temperature, humidity, cloud properties and surface winds, and thus regulates sea ice through atmospheric thermodynamic and dynamic effects. The formation of this local atmosphere-sea ice coupling is partially driven by a teleconnection originating from sea surface temperature (SST) changes in the east-central tropical Pacific via a Rossby wave train propagating into the Arctic (termed as the Pacific-Arctic teleconnection (PARC)). The forming process of the PARC (in its positive phase) and its driving force on sea ice is summarized in the following schematic diagram: The SST cooling phase in the eastern Pacific can trigger a Rossby wave train that extends from the tropical Pacific to the Arctic in a great circle, with a barotropic high-pressure system established over Greenland and the Arctic. At the surface, friction causes anticyclonic winds to diverge, leading to downward motion of air to conserve mass. As the air descends, it adiabatically warms, allowing it to hold more moisture, and, therefore, increase low boundary cloud cover through increasing relative humidity below the inversion layer. The combination of a warmer, cloudier and more humid atmosphere organized by the PARC increases downward longwave radiation, contributing to surface warming and increased sea ice melt.
This PARC mode is found to represent the leading internal mode connecting the pole to lower latitudes on multi-decadal time scales not only over the past four decades but also over a longer period going back to the past 400 to 2000 years. In particular, the PARC is believed to contribute to accelerated warming and Arctic sea ice loss from 2007 to 2012 and the occurrence of the second lowest sea ice minimum in 2020.
Based on observational features of the PARC, we further evaluate the skill of CMIP5 and 6 in replicating those key characteristics of the PARC and its local component in the Arctic. We find that the vast majority of the models exhibits low skill in replicating the PARC and its local forcing in the Arctic. This limitation appears to be due to models' biases in simulating low-frequency SST and rainfall variability over the tropical eastern Pacific and the climatological mean flow over the North Pacific during boreal summer. Considering the significant contribution of the PARC mode in shaping low frequency climate variations over the past decades and centuries from the tropics to the Arctic, improving models' capability to capture the PARC mode is expected to be essential to reduce uncertainties associated with decadal prediction and climate change projection over the Arctic in the coming decades.
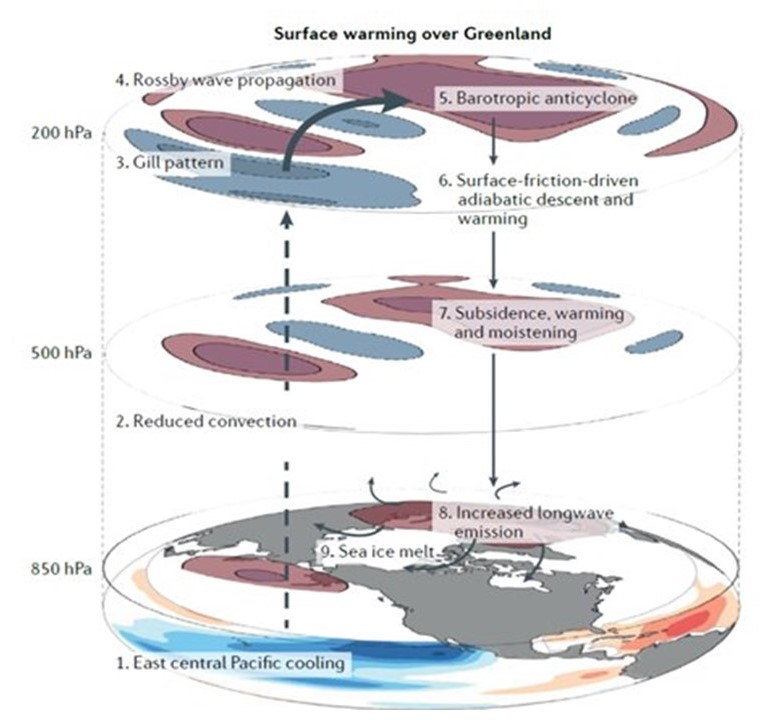
Experimental design in Ding et al. (2022)-"Nudging observed winds in the Arctic to quantify associated sea ice loss from 1979 to 2020":
Nudging wind forcing: To assess the role of the atmospheric circulation on sea ice trends, our nudging experiments nudge the CESM1’s zonal and meridional winds to observations in the Arctic’s atmosphere (north of 60°N) from the surface to the TOA. A buffer zone 2°–3° wide is set on the margin of the nudging domain to allow a smoother transition from full nudging within the domain to no nudging outside. The nudging procedure constrains simulated winds within the nudging domain to observed values at the corresponding time, by adding an additional tendency term in the momentum equations at every time step of 30 min. The 6-hourly ERA5 reanalysis observations are interpolated to model time steps (30 min) by linear interpolation. The magnitude of this tendency term is determined by a weighting parameter varying from zero (no nudging) to one (full nudging). In our experiments, this weighting parameter is selected as one (full nudging) over the entirety of each simulation to force zonal and meridional winds to vary exactly as observed within the Arctic. By doing so, the model will replay the observed circulation variability in the Arctic’s atmosphere while allowing for responses of other local systems and global climate to the specified wind changes in the Arctic.
External frocing, initial condition, Spinup and ensemble members: To exclude variability directly related to external forcings (greenhouse gases, aerosols, solar) from nudging experiments, we set external forcings as constant values at the level of the year 2000, which roughly represent the climatological mean values over the 42 years period. In addition, a long spinup run is needed beforehand to ensure that the nudging simulation has no significant “numerical shock” in the early period when reanalysis winds are imposed in the Arctic since reanalysis winds in the Arctic may be very different from the model’s own winds there. To do so, a 150-yr anthropogenic forcing-fixed (at the level of the year 2000) nudging simulation is initially conducted with the model perpetually nudged to winds of year 1979 in the Arctic (the same nudging domain and buffer zone as those used afterward). In this spinup, many key indicators of climate stability, including Arctic sea ice and pan-Arctic and global mean net radiation at the TOA and surface, start to stabilize after the first 100 years and then vary around constant levels in the last 50 years. The model states on 1 January of each of the last 10 years of this spinup are then separately used as initial conditions to reinitiate a set of new 10 members of 42-yr nudging simulations with winds in the Arctic nudged to observations from 1979 to 2020.
Monthly output of nudging simulations in Ding et al. (2022):
All following fields are the ensemble means of 10-member CESM1 nudging simulations from 1979 to 2020 (42yr) conducted in Ding et al. (2022). Daily averages of these fields are stored on Ding's local data archive and monthly averages of daily values (from 30N to 90N) are available for download. In addition, the data from individual members or other variables are available from Ding (qinghua@ucsb.edu) upon request. Please let me know if other fields are desirable.
Please cite Ding et al. (2022) when using these data.
Ding, Q., A. Schweiger, and I. Baxter, 2022: Nudging Observed Winds in the Arctic to Quantify Associated Sea Ice Loss from 1979 to 2020. J. Climate, 35, 3197–3213, https://doi.org/10.1175/JCLI-D-21-0893.1.
Field1: NH (30N-90N) monthly sea ice fraction from 1979/1 to 2020/12.
Field2: NH (30N-90N) monthly surface temperature from 1979/1 to 2020/12.
Field3: NH (30N-90N) monthly longwave radiation at the TOA from 1979/1 to 2020/12.
Field4: NH (30N-90N) monthly net shortwave radiation at the TOA from 1979/1 to 2020/12.
Field5: NH (30N-90N) monthly net longwave radiation at the surface from 1979/1 to 2020/12.
Field6: NH (30N-90N) monthly net shortwave radiation at the surface from 1979/1 to 2020/12.
Field7: NH (30N-90N) monthly geopotential height(10 vertical levels) from 1979/1 to 2020/12.
Field8: NH (30N-90N) monthly air temperature (10 vertical levels ) from 1979/1 to 2020/12.
Many more fields to come!
Key publications:
Ding, Q., A. Schweiger, and I. Baxter, Nudging observed winds in the Arctic to quantify associated sea ice loss from 1979 to 2020. J. Climate (2022)
Baxter, I., and Q. Ding, An optimal atmospheric circulation mode in the Arctic favoring strong summertime sea ice melting and ice-albedo feedback. J. Climate (2022)
Sun X, Q. Ding, S. Wang, D. Topal, Q. Li, C. Castro, H. Teng, R. Luo, and Y. Ding, Enhanced jet stream waviness induced by suppressed tropical Pacific convection during boreal summer. Nature Commun.(2022)
Li, Z., Q. Ding, M. Steele, and A. Schweiger, Recent upper Arctic Ocean warming expedited by summertime atmospheric processes. Nat. Commun. (2022)
Huang, Y., Ding, Q., Dong, X., Baike, X., Baxter, I., Summertime low clouds mediate the impact of the large-scale circulation on Arctic sea ice. Communications Earth and Environment (2021).
D. Topal, Q Ding, J Mitchell, I Baxter, M Herein, T Haszpra, R Luo, Q Li: An internal atmospheric process determining summertime Arctic sea ice melting in the next three decedes: lessons learned from five large ensembles and multiple CMIP5 climate simulations, Journal of Climate 33 (17), 7431-7454, (2020)
I. Baxter, Q Ding, A Schweiger, M L'Heureux, S Baxter, T Wang, Q Zhang, K Harnos, B Markle, D Topal, J Lu: How tropical Pacific surface cooling contributed to accelerated sea ice melt from 2007 to 2012 as ice is thinned by anthropogenic forcing, Journal of Climate 32 (24), 8583-8602, (2019)
E Blanchard-Wriggleswoth, Q Ding: Tropical and midlatitude impact on seasonal polar predictability in the Community Earth System Model, Journal of Climate (2019)
Q Ding, A Schweiger, M L'Heureux, EJ Steig, DS Battisti, NC Johnson, E Blanchard-Wrigglesworth, S Po-Chedley, Q Zhang, K Harnos, M Bushuk, B Markle, I Baxter: Fingerprints of internal drivers of Arctic sea ice loss in observations and model simulations, Nature Geoscience 12 (1), 28-33, (2019)